Antibiotic medications have a tricky job: kill the target single-celled organisms without causing so much damage to the host animal that it would be better off with the infection. Many treatments that seem promising in a petri dish can’t make that leap. So how do antibiotics work anyway?
Before the era of modern broad-spectrum antibiotics, products from the environment and early laboratories were used with varying success. An example of a product from the environment is honey. Honey creates an inhospitable environment for bacteria through several mechanisms, including changing the pH of a wound. A pH imbalance can disrupt cells in various ways, such as affecting osmolarity (the water pressure inside a cell). An example of an early product from a laboratory is dye. Dyes focus light wavelengths that cause DNA damage. As antibiotic resistance grows, the use of dyes to kill bacteria is returning to favor.
Many of us learned in grade school that penicillin was the first antibiotic. Penicillin was discovered in 1928 when a Penicillium mold growing in a petri dish in Alexander Fleming’s lab killed surrounding bacteria. Due to difficulties with large-scale production, however, it was more than a decade before penicillin became a marketed product.
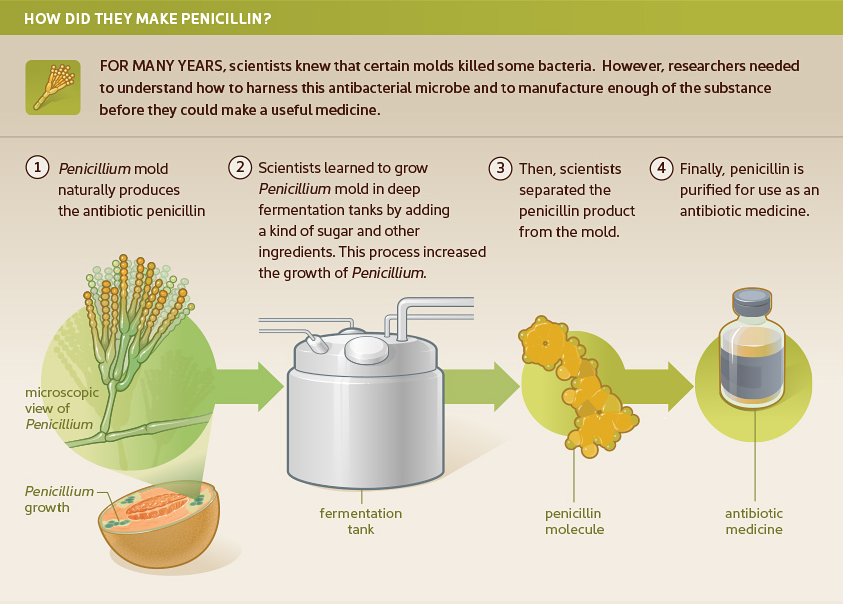
Before penicillin was available, Prontosil came to the market in the 1930s. This sulfonamide changed a world where every infection could be deadly. Sulfonamides work by preventing bacteria from creating folate (Vitamin B9), which they use to synthesize nucleic acids that make up their DNA. If they cannot create new DNA, they cannot replicate. Humans and other animals get folate from their diet, so the drug is not as deadly to them as it is to bacteria.
Penicillin did not become widely available until the mid-1940s. Bacteria multiply by splitting in half and becoming two bacteria, then four, etc. Penicillin interferes with the ability of the bacteria to build a cell wall. When a cell exposed to penicillin attempts to enlarge the cell wall in preparation for division, instead it burns open and dies. This antibiotic only works on certain kinds of bacteria and only works when they are dividing. Therefore, for slow-growing stealth pathogens, like Bartonella species, this antibiotic would not be very effective.
The race to find more antibiotics heated up in the 1950s, when tetracycline antibiotics were discovered in soil samples and developed into drugs. Doxycycline is a tetracycline. Tetracycline drugs interfere with the ability of the bacteria to create the proteins it needs to survive. Tetracycline antibiotics can only get through the cell wall of certain kinds of bacteria, so they damage animal cells much less than the cells of certain kinds of bacteria. Tetracyclines were a game changer for deadly infections like Rocky Mountain spotted fever that had previously resulted in high fatality rates.
Tetracycline also increases growth in animals for reasons that are not completely understood. For this reason, it was added to animal feed. Combined with overuse in medical treatment, bacteria developed resistance by forming new kinds of proteins that are not affected by tetracycline.
There are many types of antibiotics, but two others that are often used in the treatment of tick-borne diseases are from the rifamycin and quinolone groups:
- Rifamycins, of which Rifampin is one, came from a chemical isolated from bacteria discovered in a soil sample. As pharmaceutical companies had success with soil samples, they collected samples from all over the world to find new antibiotics. Rifamycin interferes with the ability of the bacteria to make proteins. The exact place that it interferes with production is different in mammalian cells, so the cells of the host are not damaged. Unfortunately, it requires the change of only a single amino acid to make the bacterial cell like the mammalian cell, so bacteria easily become resistant to rifamycin.
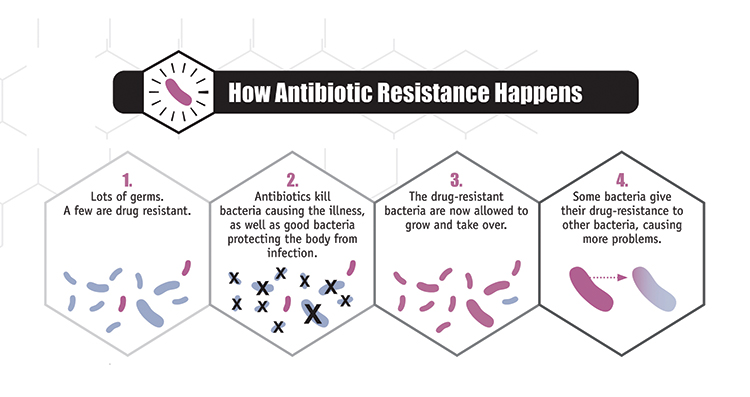
- Quinolones had been in use for a long time, but fluoroquinolones were latecomers to the antibiotics race, arriving in the late 1980s. These antibiotics were discovered in a laboratory. When a cell divides, it makes complete copies of its DNA to go to each subsequent bacterial cell. Fluoroquinolones interfere with that process. The enzyme they target is different in different kinds of bacteria and in mammals, so it can harm certain bacteria while not causing the same kind of harm in the host.
Conclusion
As the golden age of antibiotics passes, new strategies are coming on board. For example, methods that utilize CRISPR technology are becoming popular because they can be used to target and destroy specific segments of bacterial DNA. The ever-present challenge is how to damage bacteria while ensuring that the animal host is not damaged in the process. As the importance of the microbiome is recognized, that challenge now includes how to preserve the other bacteria that make up the microbiome.
References
Gould, K. (2016). Antibiotics: From prehistory to the present day. Journal of Antimicrobial Chemotherapy, 71(3), 572-575. doi:https://doi.org/10.1093/jac/dkv484 https://academic.oup.com/jac/article/71/3/572/2364412
Mandal, M. D., & Mandal, S. (2011). Honey: Its medicinal property and antibacterial activity. Asian Pacific Journal of Tropical Biomedicine, 1(2), 154-160. doi:10.1016/S2221-1691(11)60016-6 https://www.ncbi.nlm.nih.gov/pmc/articles/PMC3609166/
Sensi, P. (1983). History of the development of rifampin. Reviews of Infectious Diseases, 5(Suppl3), S402-S406. https://www.ncbi.nlm.nih.gov/pubmed/6635432
Wainwright, M. (2017). How we can use light to fight bacteria. The Conversation. Retrieved from: https://theconversation.com/how-we-can-use-light-to-fight-bacteria-73036